Disclaimer: This article originally appeared in the March 2021 issue of Cannabis Science & Technology.
Authors: Robbie Batts, InSpire Transpiration Solutions and Samuel Burgner, PhD Candidate, McGill University
Why should leaf temperature matter to you as a cannabis cultivator? There are many reasons to consider, but the answer starts with a solid grasp of the basic concept of temperature.
Heat is a form of energy and temperature is a measure of that energy. When you are outside and feel the warmth of the sun on your skin, it’s not the same as standing around a fire absorbing the heat it emits. The sun releases large quantities of infrared radiation, which is a form of electromagnetic radiation better known as light. Infrared radiation on its own is not “hot,” but when it intercepts objects like your skin or a plant leaf, it is absorbed. This causes the molecules to vibrate at a higher frequency (more energetic), generating heat and consequently increasing the temperature.
This is why you can feel intense warmth when standing in the sun and then instantly feel cold when a cloud covers the sun. The radiative infrared light source has been intercepted by clouds (water), which absorb infrared very strongly.
Plants and infrared radiation
Plants are full of water, so they are very susceptible to absorbing infrared radiation. When you cook food in a microwave, water molecules in the food absorb the microwave radiation, making the molecules more energetic and generating heat to cook the food. This is similar to how infrared radiation causes plants to become warmer.
It is important to understand the different molecules that make up a cannabis plant in order to grasp the influence of infrared exposure. The majority of carbon matter in a cannabis plant (such as cellulose, the fibers that make up most cell walls and structures like stems and leaves) does not effectively absorb infrared radiation, nor can it retain much heat. However, water molecules in plants strongly absorb infrared, creating an energetic stream of fluid moving through the stems and vascular tissue.
Water has a high capacity for absorbing heat, meaning that it takes a lot of energy to raise the temperature. Water also tends to absorb heat from warmer objects in its immediate surroundings due to this intrinsic property. Consider the difference in temperature between a slab of concrete sitting in the sun versus the puddle of water that has collected from recent rainfall.
How plants use heat
Heat is moved into a plant in three primary ways: conduction, convection or radiation.
- Conduction is when heat is transferred by touching. When an object is hot, it conducts heat into the air immediately near it. In horticulture, the impact of conduction is primarily in the root system where the plant is physically touching something else (soil, water, etc). This is why rootzone heating is so effective – water in the soil absorbs the heat energy and disperses it throughout the cannabis plant.
- Convective heating is when air containing heat energy is moved around, transferring its heat energy to cooler air and objects in the room. Picture a high-pressure sodium (HPS) fixture with a fan directed at it. The air in the room would conduct heat from the HPS fixture and generate a convective current as that heat energy in the air is moved throughout the room (technically called forced heat convection). The convective heat is contained by the air molecules, which are then moved by the fan, resulting in a net increase in temperature in the room as more of this heat energy is emitted by the HPS fixture.
- Most of the heat in a commercial cannabis grow room comes from radiation emitted from the light source and all surfaces. All of this energy is constantly released and absorbed by objects or plants in the room at the speed of light as infrared radiation. This matters most when the lights are turned off in a grow room and the room is kept warm by radiating surfaces until enough energy has been lost to drop the temperature below setpoint. At this point, the climate control system will need to provide heat.
All objects emit infrared radiation when they are above absolute zero temperature and if the temperature is high enough, they will emit visible light – like coals in a fire. Unless airflow in the room is very high or rootzone heating is being used, most of the heat energy absorbed by a plant will be in the form of radiation.
Plants’ tolerance range for temperature swings
All plants have range of heat tolerance based on a variety of factors:
- Cannabis transpiration rates will increase if the canopy temperature increases because the energetic state of the water in the plant is higher, making the molecules move more quickly and increasing vapor pressure within the leaf (this will be covered in our next article).
- Most of the protein in a cannabis plant’s leaf tissue is in an enzyme called Ribulose Bisphosphate Carboxylase Oxygenase (RuBisCO). This enzyme is responsible for capturing carbon dioxide gas using energy produced by photosynthetic light absorption. RuBisCO can bind carbon dioxide and oxygen. If the temperature is too high (oxygen solubility increases), the enzyme becomes prone to binding oxygen instead of carbon dioxide. This ends up burning the plant’s stored energy to carry out this process, resulting in slower growth. This process, known as photorespiration, is totally dependent on leaf temperature as opposed to room temperature.
- All of the contents of a plant are confined in its cells using fat-based membranes. As we know, some fats are solid at low temperatures, while others remain liquid. Those that remain liquid can become extremely fluidic at high temperatures. The fats that make up the cell membrane will change in response to temperature to ensure that a solidifying fat is not employed at low temperatures (which would “crack” the membrane as its movement slows, making the cell leak), or that a very fluid fat is not used at high temperatures (which would become too fluid and also result in a leak). When temperature changes, it can take several days for the plant to alter its cells to deal with these changes and each genotype is limited by its capacity to alter these membrane structures. This is part of the reason we have seasonal crops. If the environment shifts rapidly and frequently, the plant does not have enough time to adapt and can be damaged.
These factors constitute the tolerance range plants have for temperature swings. Fortunately for cultivators, cannabis plants are quite adept at making up for this stress. Stomata – the pores found primarily on the underside of leaves – release water from the inside of the plant into the atmosphere and can open and close to regulate how much moisture is released from the plant. Plants take up water in the rootzone, which is then moved through the vascular tissue and into the stem, which eventually enters the leaf cells where it accumulates on the outside of the cells in the intercellular air space. Once it reaches this point, water evaporates into the leaf air space, where it can exit the plant through the stomata.
What is the mechanism that causes this evaporation and what are some of the consequences? When water evaporates, it takes the heat energy with it, resulting in a net cooling effect for the object from which it came – in this case, the leaf. As water moves through the vascular tissue in a plant, it also absorbs heat from its surroundings. By the time water reaches the leaf, the energy provided by the lights plus the pull on the liquid surface imparted by the atmosphere will provide the last bit of energy needed to change its state. At this point, water becomes vapor and shifts into a dynamic with the atmospheric conditions outside of the plant. Aside from keeping the leaf inflated, the water has done its job and room conditions outside of the plant promote or inhibit its ability to escape the leaf at a healthy rate without smothering or drying the plant.
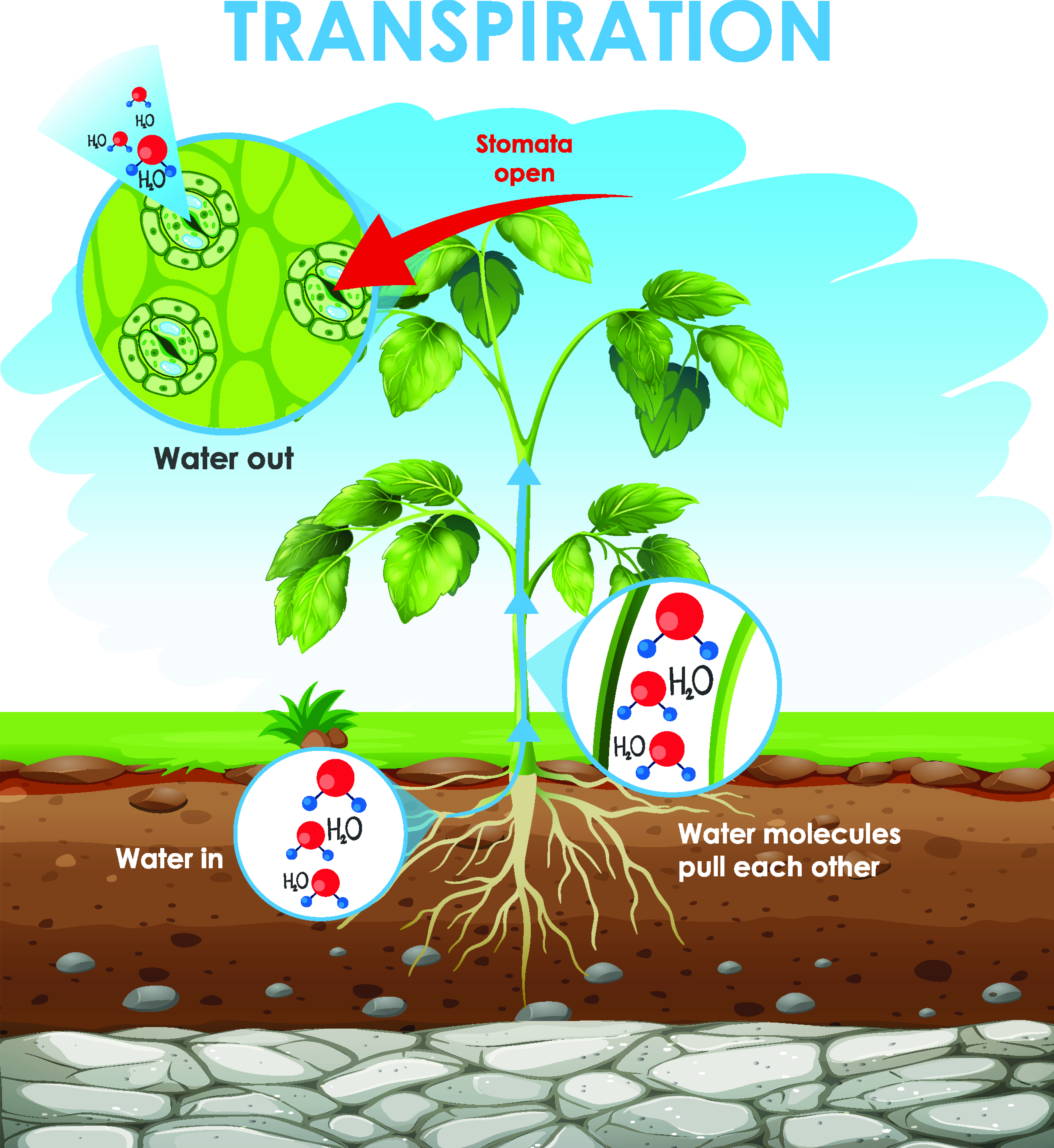
Leaf temperature and plant growth
Respiration rate is another plant growth process that provides most of the energy-rich compounds cannabis plants need to grow and also what the plant needs to survive when the light source is gone. A large portion of respiration occurs in the roots, where plants tend to store captured photosynthate. If the temperature of the leaf or root is too high, the growth respiration rate may be higher, but the amount of energy the plant will spend on maintenance respiration might outweigh any potential gains. This is part of the reason many growers choose to use cooler temperatures at night.
Leaf temperature also impacts leaf expansion, which is critical for ensuring light capture. As the temperature rises in the plant, pressure builds up and forces the cell walls to stretch and grow. If the leaf temperature is too high, however, the plant will focus energy on cooling itself by opening its stomata, increasing transpiration rates and leading to a pressure drop that reduces the amount of expansion. By providing a slightly cooler temperature and a higher humidity level at night, roots can build up additional pressure. This forces water into the tissues of the plant, leading them to grow and evenly distribute nutrients to both mature and developing tissues.
Plant tissues can be at different temperatures within the same grow room. The roots, stems and leaves can all vary in temperature, and this difference is exacerbated by proximity to the light source and access to air movement. With plant tissues at different temperatures, maintenance respiration rate, growth rate and expansion can all vary.
In conclusion
By grasping the concepts discussed in this article, cultivators will have a better understanding of how to balance the temperature of cannabis plants to reach maximum growth rates. The optimum leaf temperature changes depending on how much light intensity and carbon dioxide are supplied to the plant. Light intensity and carbon dioxide are captured at faster or slower rates depending on the temperature of the proteins involved. All factors must be in careful balance to determine the optimum leaf temperature.
Our next article in this series will dive deeper into water movement in plants, primarily focusing on the concept of vapor pressure deficit (VPD) and VPD control.